Clean Tech Will Do Far More Than Stop Climate Change
Terraforming, material abundance, and bountiful energy
The full impact of a new technology is rarely recognized at the time. What surprises people are not fundamental changes to the technology, but the opportunities unlocked by scale.
The semiconductor fabrication industry has scaled chip density by honing the same techniques for 30 years, but nobody could’ve predicted that with an enormous amount of data and an enormous amount of computation we could teach those chips to think1.
This is where my mind goes when people talk about using desalination to provide drinking water. It’s like thinking about computers as calculators; an important application, but it misses the point.
Clean technologies aren’t just tools to save the world from our own flatulence, they have the potential to reshape life as we know it. As we’ll see, advances in renewable energy, batteries, desalination, electrolysis, and carbon capture can reshape society, just as fossil fuels once did.
Renewable Energy
Fortunately, renewable energy has rapidly declined in cost, making it the cheapest source of energy in most places on earth. Cheap energy enables the other technologies in this post.
Having a cheap but intermittent source of energy will change how most processes are structured. For example, since solar energy is only available in the daytime, why not run energy-intensive industries like steel-making during the day? As we’ll see later, many chemical processes can take advantage of cheap electrons from solar and wind2.
In this context, I wonder how data centers and supercomputers will adapt to renewables. Could large AI training runs jump from cluster to cluster, running in the sunlight to save on energy costs?
Semiconductor fabrication might also maneuver to take advantage of cheap energy. Fabs require lots of energy for lithography and energy-intensive resource inputs like ultrapure water. Along with the fact that this process needs input from highly skilled technicians operating during daylight hours, switching to renewables is a natural next step.
Transitioning industries over to renewable energy inputs will take a long time. Each industry will have to reorient around a temperamental but abundant power source. At the end of this painstaking process, we will see cost reductions for virtually every product and a simplification of manufacturing systems.
Batteries
Batteries can help with the intermittency of renewable energy, at a cost. If they get cheap enough, we can shift most of our energy production to wind and solar, effectively localizing energy production and eliminating reliance on the grid. Many households sell rooftop solar back to grid operators, with cheap storage, they can keep it for themselves. This will make communities more resilient to power outages.
Batteries are also getting good enough to displace fossil fuels as an energy source in cars and trucks. Air pollution from driving sickens millions of people each year, so electrifying transportation will have a big impact on human welfare.
One of the last modes of transportation to be electrified will be flight. It’s not clear if it will ever be economical compared to fuels, but I’m optimistic. There are some battery designs that get close to fuel’s energy density, and electric motors are lighter and more efficient, closing the gap. Electrifying passenger flights wouldn’t just make air travel greener, it would enable numerous small flights and flying cars, making air travel available to everyone on a daily basis.
Desalination
The energy costs of desalination have fallen from 30 kWh/m3 in 1970 to less than 3 kWh in 2018, with the potential to fall as low as 1 kWh/m3. This means that producing desalinated drinking water now has a trivial energy cost, as Anna-Sofia Lesiv points out:
Given that the average American household consumes somewhere around 100,000 gallons of water per year, the total energy required to desalinate that much water through reverse osmosis would be something like 1,135 kWh per year. That’s less than the average annual energy consumption of the typical refrigerator — which sits somewhere around 1,400 kWh.
But that American household doesn’t really care where their water came from; drinking water is cheap in rich countries. Thinking about desalination as a way to produce drinking water is thinking too small; instead, desalination can be used to produce massive quantities of freshwater3.
For example, Casey Handmer points out that:
Artificially supporting the Colorado river’s flows in their entirety would cost only a few billion dollars a year – not even cents on the dollar compared to its economic productivity. It is true that the Colorado is not a large river by global standards, but if we’re facing global water shortages we should be happy to have effectively infinite extremely cheap solar power available to re-irrigate what limited arable land we must depend on.
By my math, replacing the Colorado river with desalinated water would cost less than $2 billion/year, or less than $40 per person living in the river basin4. Even at 10X the cost, it’s still a bargain.
This is why cheap solar energy is so important. At the scale required to replenish rivers, energy costs become significant even when desalination is efficient. But cheap solar energy makes these costs manageable. And because of how much solar energy hits the earth, huge transformations are possible. To give you a sense of scale, the solar energy hitting the Sahara desert can desalinate the Mediterranean sea in less than 5 years5.
With a little investment, solar and desalination can provide clean water to drought-stricken communities, replenish rivers, and fix ecosystems damaged by climate change6. But we can go further, freshwater is fundamental to all life on earth and producing it in abundance means that we can create new ecosystems. Even with a little seawater, deserts can bloom with new life. With enough water, any arid region can become green. I personally would love to see an abundance of fresh water used to create jungle-like ecosystems at any latitude. Imagine hiking though the Amazon in Arizona!
Freshwater can also be used to control local climates. Nearby bodies of water tend to moderate temperatures, and large-scale irrigation tends to prevent high temperatures nearby.
Desalination comes with one environmental concern: what do we do with the brine? Fortunately, desalination brine creates more opportunities than problems. For one, brine electrolysis is used to produce important chemicals, and many people are interested in extracting precious metals from the sea. The sodium can be used for sodium-ion batteries, and companies like Magrathea metals are hoping to use the magnesium to make electric cars. While we’re at it, we might as well scrub the carbonates from brine to fight acidification and use the dissolved magnesium and calcium to capture CO2.
Green Hydrogen and Electrochemistry
With renewable energy and desalination, we can produce fresh water from the sea in massive quantities. With another zap, we can chemically transform that water.
Splitting water into hydrogen and oxygen has been studied for centuries. But only recently has it received massive amounts of investment as a clean technology, able to replace fossil fuels in many chemical processes.
The key is cheap hydrogen production is to re-orient the process to take full advantage of renewable energy. Put solar panels right next to your electrolyser and run while the sun is shining to eliminate energy transmission costs. Then feed that hydrogen directly into another chemical process to eliminate hydrogen storage costs. Casey Handmer thinks we can use these tricks to get below $1/kg of hydrogen, enough to make electrolysis competitive with fossil fuels.
What can we do with cheap hydrogen? Lots of fun things. Since hydrogen is a lifting gas, we can use it for airships, aerostats, and sunshades7. But hydrogen is far more exciting as a chemical reagent. Many chemical processes need hydrogen to transform inputs into useful products.
Take nitrogen for example. We take nitrogen from the air and combine it with hydrogen to produce ammonia, the chemical precursor to all fertilizers. This is known as the Haber-Bosch process, and it’s the reason you’re alive today; roughly half of the nitrogen atoms in your body were produced with Haber-Bosch. Today, this process uses natural gas as the hydrogen source, and the cost of natural gas drives the price of fertilizer. If green hydrogen gets cheap enough, it could lower fertilizer costs and simplify the production process. Several companies such as Nitricity, Jupiter Ionics, Flemtec, and Atmonia are researching how to produce fertilizers using renewables8.
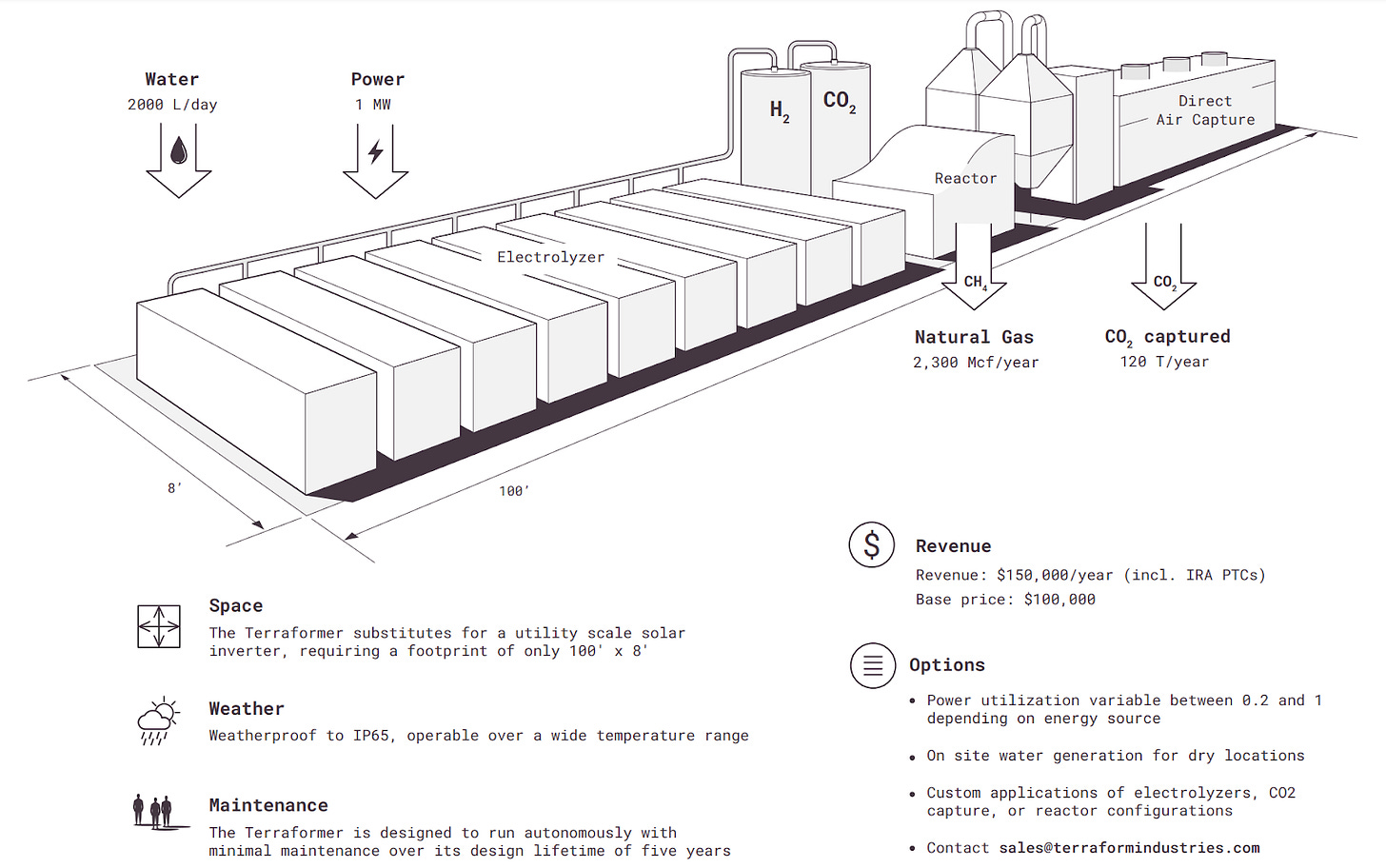
Hydrogen is also important for processes like steel making, CO2 reduction, and fuel synthesis. Deriving hydrogen from water means that all of these industries can lower costs and eliminate their reliance on fossil fuels.
Hydrogen isn’t the only chemical that benefits from renewables. The entire field of electrochemistry is trying to figure out how to use cheap, renewable electrons as a chemical reagent9. Inorganic materials will also benefit. Several companies are trying to produce aluminum, magnesium, steel and concrete by leveraging renewable energy. Electrometallurgy used to refine ores may also benefit10.
In every case, renewables can make these processes simpler and cheaper, building on the material abundance created by fossil fuels, without the side-effects.
CO2 Capture
CO2 capture has been put forth as a way to reverse centuries of carbon emissions, with massive effort being invested in lowering the cost per ton of CO2 captured. But what are we going to do with billions of tons of carbon?
We could try to inject it into the ground or turn it into rocks, but that’s boring. Carbon is the backbone of all organic matter, so let’s make something with it. Captured CO2 can provide the carbon source that fossil fuels provide today. Companies like Terraform Industries, Prometheus fuels, and Twelve are jumping on this opportunity, turning captured CO2 into chemical products.
The interesting thing is that CO2 is evenly distributed across the world. Since shipping adds costs, many chemical processes will switch to a local production mode, using renewables and air to make pharmaceuticals, plastics, and food. Soon, every nation will have secure access to the products needed to support modern life.
At a large enough scale, we can adjust the CO2 concentration of our atmosphere giving us control over the global thermostat. In the future, an international body might set goals for CO2 concentration in order to boost crop growth or change the weather.
Conclusion
Fossil fuels are a fantastic energy source, but mining them, moving them, and transforming them into energy requires a lot of moving parts. Replacing all of that with the humble solar panel will reshape every industry.
Solar, desalination, batteries, and electrochemistry will continue to extend their lead over traditional chemical processes. But the real advantage lies in applications that combine these breakthroughs in new ways. Together, they imply the ability to synthesize organic matter out of thin air. How will the world change when people can make food, fuel, polymers, and medicine in their own backyard11? More generally, people can use local energy sources to transform the matter around them, effectively decentralizing the atom.
Take a moment to consider the geopolitical implications. When anyone can make fuel, fossil fuels will lose their power over international politics, lifting the resource curse and bolstering democracy. It will also reshape international trade as many chemical processes shift to local production12.
I find it heartening that some of the biggest winners from clean technologies will be people in Africa. Trade to the interior is prohibitively expensive due to the continent’s size, but since it’s awash in solar energy, people in remote regions might make their own way, producing fertilizer and fuel themselves. Many people in Africa lack access to a reliable electrical grid or running water, yet clean technologies tailored to their needs would make these things superfluous.
I’ve seen extraordinary technological developments in my lifetime, from genome sequencing to reusable rockets to artificial intelligence. But of any of them, clean technologies give me the most visceral sense of progress. We quietly gained the ability to terraform the earth; everything else pales in comparison.
Further Reading
Casey Handmer has written a lot about what the future of energy and chemical production looks like. A few relevant pieces:
We’re going to need a lot of solar panels
The future of electricity is local
The Unstoppable Battery Onslaught
Grid Storage: Batteries Will Win
How to Produce Green Hydrogen for $1/kg
We need more water than rain can provide: refilling rivers with desalination
Austin Vernon has many interesting pieces relating to clean technology. A few examples:
The Decentralization of Atoms is Underrated
The Future of Industrial Process Heat
The Future of Carbon Dioxide Direct Air Capture
Electric Trucks are Ready for the Road
Simple Solutions Power Solar's Advance
Energy Superabundance: How Cheap, Abundant Energy Will Shape Our Future (coauthored with Eli Dourado)
Tomas Pueyo:
Other:
Can We Terraform the Sahara to Stop Climate Change?
Atmospheric Water Generation Technologies
I created a plate on vienna.earth to visualize what I call Solar Ocean Material Abundance: using solar along with the resources of the ocean to create a new way of life.
Eliezer Yudkowsky claims that “[n]obody called LLMs, as far as I know.” So far I haven’t seen anyone reply with a link to a prediction. Some people predicted that AI would arrive around this time, but not the specific development path of language models.
Renewables can even provide high-quality industrial process heat.
Desalination isn’t the only way to produce fresh water, atmospheric water capture is also being developed. This guy wants to develop cloud seeding technology to generate more freshwater.
Flow in the lower basin is 9 billion m3/year, desalination costs 2 kWh/m3, and renewable electricity costs $30/MWh. This cost of renewable energy is multiplied by a factor of 3 relative to what I expect in order to include the cost of pumping the water to an appropriate height (though some of this energy will be recouped hydroelectric generation). Handmer suggests we multiply by another factor of 3 because we will want to overbuild solar capacity so it can run entirely during the day. Multiplying out, this becomes $1.6 billion/year.
The Sahara desert receives 22 billion GWh of sunlight each year. Lets assume a solar conversion efficiency of 10%, and a desalination system that requires 2.0 kWh/m^3 of water. The Mediterranean sea is 4.4E15 cubic meters, so that comes out to roughly 3.5 years of solar energy to desalinate it.
Access to freshwater may make seasteading more viable and enable water-intensive crop growth, food production, fermentation, ultra-pure water production, etc. We can also turn every briny sea into a freshwater lake and terraform every island.
Some other uses of hydrogen balloons include sunshades, telecommunications, city-wide parasol’s, and launching platforms for flying cars and drones.
I also wonder if something like the Birkeland-Eyde process could make a comeback using renewables. Nitricity’s approach is most similar to this.
There’s also an approach to solar fuels that uses sunlight to directly drive chemical reactions. See Sophia Haussener’s work and JCAP.
Personally, I wonder if we can electrify the purification of silicon, the way it’s made today isn’t fitting for our high-tech era.
One concern I have is that these capabilities allow anyone to produce explosives in their backyard, which may increase the risk of domestic terrorism and require ubiquitous bomb-sniffing technology.
Fuel also plays an important role in military strategy; if navies can produce fuel at sea, it may change naval strategy significantly. I would guess that this would make nations more peaceful, as there are less resources that war would justify and less of a mismatch in resources between countries.
You're probably not going to run a semiconductor fabrication plant and/or AI training intermittently. These are very expensive machines and hence you want to maximise utilisation even if you have to pay more for electricity. Utilisation is a big deal for capex heavy industries.
I doubt green hydrogen will be as big of an industry as the pundits are making it out to be. Solar electricity to hydrogen to heat is an extremely inefficient process. Transport and heat will mostly be electrified. You can efficiently convert electricity to heat and store that heat in heat batteries for months at very low losses.
Hydrogen is mostly be used a feedstock for making chemical like plastics. Hydrogen will not be a big player in the steel industry. When electricity is cheaper than heat, more industrial processes will shift from thermochemical systems to electrochemical systems as demonstrated by companies like Boston Metals.
Great article. One of the few business leaders who seems to recognize the interplay between ideas and scale is Elon Musk.
The Starship rocket, which I have written about a few times, is intended to be the world’s first fully and rapidly reusable rocket. But Musk and his engineers recognize that being reusable is not enough, launch frequency, or scale, is (actually) more important.
Just as a 737 would not be economical to fly once a month, even if it wasn’t expended, a rocket cannot be grounded for weeks at a time. To make space access affordable, rockets have to become like airplanes…always launching and landing. He then extends this interplay to rocket design and production. The more frequently a rocket flies, the more opportunities engineers have to identify failure modes and improve the design to scale up reliability.
Doing this requires rapid production and iteration, so he has built a rocket factory that hopes to churn out Saturn V-sized rockets once every few days. What SpaceX, at Musk’s behest, is borderline insane. It’s rational madness that is built on an understanding of scale.